Bíró Szabolcs állításából ezt szűrheti le az olvasó:
Olyan készítményeket érdemes keresni, amelyekben a B5-vitamin pantetin formájában van, ami a B5-vitamin jobb/aktívabb formája, mint a pantoténsav (pl.: Ca-pantotenát).
Bíró Szabolcs indoklása:
A Pantetin panteteinre bomlik, ami sejten belül már több lépéssel közelebb van a végső, aktív Koenzim-A (CoA) formához, mint a pantoténsav/pantotein, így az könnyebben alakul CoA-vá. Ennek igazolására 1 db szakcikket meg is ad referenciának, azonban abban csak megemlítik a pantetint, mint ami esetleg hatásos lehet azoknál, akik a sima B5-vitamint (pantoténsavat) nem tudják átalakítani (7 évvel később egy vizsgálat rácáfol erre, de már előtte lévő vizsgálatok is indirekt rácáfoltak).
Az én állításom:
A pantetin, egy élelmiszereinkben elő nem forduló B5-vitamin analóg, amely 10x drágább, de maximum azonosan jó, mint a sima pantoténsav/pantotenát (pl.: Ca-pantotenát), mivel szájon át szedve eleve 100%-osan átalakul pantoténsavvá, mielőtt a véráramba jutna, a hasznosulása pedig kevésbé jó, mint a pantoténsavnak (pl.: Ca-pantotenátnak). Farmakológiai/szuprafiziológiás (óriási) dózisokban alkalmazva a pantetin rendelkezik olyan hatásokkal, amelyekkel a B5-vitamin nem rendelkezik semmilyen dózisában. A pantetin ezen hatásai azonban nem a B5-vitaminná való alakulásának képessége miatt, vagy a B5-ből képződő aktív koenzim (CoA) szintet emelő képességéből adódnak, azaz nem abból, hogy „aktívabb” vagy jobban hasznosuló B5-vitamin forma lenne, hanem abból, hogy ciszteaminná bomlik a szervezetben. B5-vitaminként a pantetin használata semmiképpen sem előnyös a mezei pantoténsavhoz képest. A pantetinnek tehát van ugyan a B5-vitamintól eltérő terápiás hatása szuprafiziológiás/farmakológiai dózisokban alkalmazva, azonban B5-vitaminként való használatának maximum nanokolloid rendszer részeként, vagy esetleg beinjekciózva lenne csak értelme, óriási dózisban adagolva, ugyanis szájon át szedve előbb alakul át sima pantoténsavvá a vékonybélben, minthogy a véráramba jutna. A pantetin használata mellékhatásmentes ciszteamin forrásként sok esetben jó hatású (pl.: koleszterin csökkentés céljából), de B5-vitamin forrásként nincs helye készítményekben. Nem baj, ha van bennük, de csak fölöslegesen drágítja őket, így csupán marketing szerepe van.
Az én indoklásom:
Élelmiszerekben a B5-vitamin főleg Koenzim-A (CoA) és 4-foszfopantetein formájában van jelen, és csak kis részben pantetein és pantoténsav formájában. [1,3,5,6] A CoA a már kész, „legaktívabb” formája a B5-nek, azaz ő maga a koenzim, a végső forma, ami a B5-ből alakul a sejtjeinkben. A 4-foszfopantetin pedig 2 lépéssel a CoA előtt lévő formája. Hasonlóan tehát a B1-, B2- és B3-vitaminhoz, élelmiszereinkből a B5-vitamint is már főleg abban a formában vesszük magunkhoz, amivé később a sejtjeinkben alakulnia kell, azaz a kész koenzim formájában. Ez természetes, hiszen amit elfogyasztunk, az is élőlény, és azért vannak bennük ezek a vitaminok, mert nekik kell (az ő sejtjeiknek), nem nekünk termelik őket, az ő sejtjeik is így, a kész formáikban használják őket. Mi azonban nem tudjuk ezeket hasznosítani közvetlenül, nem kerülnek csak úgy be az elfogyasztott élelmiszer sejtjeiből a mi sejtjeinkbe. Először az emésztőrendszerünkben szét kell bontanunk őket a legegyszerűbb formáikra, hogy a bélsejtjeinken keresztül átjuthassanak a véráramunkba, majd szisztémásan a sejtjeinkhez a vérárammal eljussanak, és a sejtjeinkbe bekerülhessenek. A B1 esetében ez a felszívódni/hasznosulni/sejtekbe bekerülni képes forma a tiamin, a B2 esetében a riboflavin, a B3 esetében a niacin/niacinamid, míg a B5-vitamin esetében ez a pantoténsav/pantotenát. Először tehát lebontjuk a már kész formákat a legkezdetlegesebb/legegyszerűbb/legkisebb formára, hogy eljusson és bejusson a sejtjeinkbe, amikben aztán újra felépül a legegyszerűbb formából a kész forma. Az alábbi ábra mutatja ezt jól a B5-vitamin esetében.[1] (A piros nyilak az élelmiszerekből származó kész formák lebontásának útját mutatják pantoténsavvá/pantotenáttá, míg a zöld nyilak a kész koenzim forma sejten belüli felépítésnek lépéseit pantoténsavból).
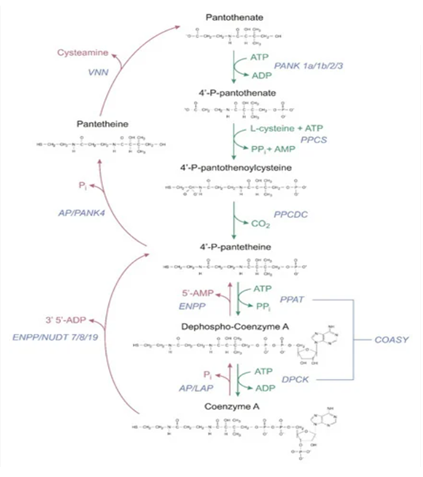
Étrendkiegészítőkben a B5-vitamint nátrium- vagy kalcium-pantotenát formájában szokták alkalmazni, amik lenyelve vagy bármiben feloldva azonnal nátriumra illetve kalciumra és pantoténsavra bomlanak (disszociálnak). A dexpantenol egy másik forma, de annak inkább krémekben van helye, így arra nem térek ki. A pantetin pedig egy újabban engedélyezett formája, amely a B5-vitaminnak egy tulajdonképpen mesterséges analógja, ugyanis élelmiszerekben nem fordul elő. 2 db pantetein molekula képez 1 db pantetin molekulát. A pantetein egy élelmiszerekben is előforduló B5-vitamin forma. A pantetin a lenyelés után a vékonybelünkben a felszívódása előtt panteteinné, majd sima pantoténsavvá (pantotenáttá) bomlik. [1,3,4,5,6] Részben már a vékonybélben, az ott bőségesen jelenlévő panteteináz enzim által lebomlik pantoténsavvá, míg egy része panteteinként vevődhet ugyan fel a bélsejtek által, amennyiben nagy a dózis, és a bél lumenben nincs elég enzim az átalakításához, azonban ez esetben a bélsejtekben bomlik le az általuk felvett pantetin pantoténsavvá. [5,6] A véráramba végül kizárólag pantoténsav formára bomolva kerül be a pantetin is. Ezt emberekben is és állatokban is vizsgálták. Embereknél egészen extrém dózisban adagolva a pantetint (1000 mg /kg) sem sikerült a véráramba akár csak nyomokban eljuttatni, csakis pantoténsavvá alakulva jelent meg a véráramban. [1,4] A pantetin és a Ca-pantotenát hasznosulása egy másik vizsgálatban össze is van hasonlítva. [6] A pantetinnek maga a felszívódása egy picit ugyan jobbnak tűnik, azonban gyorsabban ürült, így végeredményben a Ca-pantotenát dózisához képest 2x-es pantetin dózis is csak 18%-kal növelte jobban a B5 vérszintet, amiből azt állapították meg, hogy bár a pantetinnek is jó a hasznosulása, az elmarad a Ca-pantotenátétól, vagy maximum azonos. [6]
Mindebből egyértelműen következik, hogy a pantetin orálisan használva nem jelenthet előnyt a sima pantoténsavhoz képest, csak hátrányt a CoA szint növelésében, azaz a B5-vitamin hatását tekintve. Ennek ellenére mégis volt humán klinikai vizsgálat, ahol a pantetin nagy dózisának szájon át való szedését tették próbára, hátha hatásos lesz a CoA szint növelésére ún. PANK mutációval rendelkező gyerekek esetén. [2] Ez egy olyan genetikai probléma, ahol a pantoténsavat nem tudják a sejtek átalakítani az aktív CoA-vá, egymillióból egy-két embert érint, és egy PKAN nevű idegrendszeri betegséget okoz. PANK mutációval rendelkező muslincák pantetinnel való kezelése áthidalta ezt a konverziós problémát, emiatt próbálták ki embereknél is. [2] Felteszem, egyszerűbb volt szájon át szedve alkalmazni, és ezért nem intravénásan alkalmazták, bár ez számomra elég meglepő, mert már a korábbi vizsgálatokból tudhatták volna, hogy szájon át szedve nincs előnye a sima Ca-panotenáthoz képest. Nem is volt:
A PANK defektussal rendelkező gyerekek fél éven át napi 60 mg /kg pantetint kaptak (igen masszív dózis, 60 kg-os felnőttre átszámolva ez ugyanis 3600 mg /nap). A várt CoA szint növekedés elmaradt, alacsonyabb lett a vizsgálat végére, mint a kezdetekor. Elképzelhető ugyan, hogy segített mérsékelni az állapotuk romlását, azaz a CoA szintjük csökkenésének ütemét talán fékezhette, no de azt a sima pantoténsav (pl.: Ca-pantotenát) is fékezte volna, hiszen nem nulla PANK enzim-defektus esetén sem csak a konverzió hatékonysága rossz.
A Vitaminipar könyvben megadott referencia [Zorzi 2013] annak alátámasztására, hogy a pantetin áthidalja a konverziós problémákat, és emiatt jobb, mint a pantoténsav, egy olyan szakcikk volt, amelyben csupán egy pár soros említés szerepel a pantetinről: pont a PANK-enzimdefektusos muslincás vizsgálatot említik, hogy ennek fényében egy érdekes alternatív kezelés lehet a pantetin PANK defektus estén, hiszen a muslincáknál az képes volt CoA-vá alakulni, így érdemes lenne ezt a témát embereken is megvizsgálni… Ennyit írtak benne. Nyilván Zorzi és társai sem arra gondoltak ezzel a cikkükben, hogy szájon át adva lehetne hatásos… Alighanem a pantetin valóban hatásos is lenne PANK mutáció esetén vagy bármilyen esetben, ahol a pantoténsav->CoA konverzió sérült, csupán nem orálisan kellene beadni, hanem intravénásan, vagy még inkább nanokolloid rendszer részeként (pl.: liposzómák), így ugyanis el lehetne juttatni a sejtekhez és egyben a sejtekbe is változatlan formában, pantetinként, ami aztán panteteinné bomlana a sejtekben, ahol viszont már nem tudna visszabomlani pantoténsavvá, mivel a sejtekben belül már nincs ehhez enzim [1], így csak „előrefelé”, a CoA irányába tudna alakulni, azaz így valóban több lépéssel előrébb lenne, mint a pantoténsav, és áthidalhatná a problémát embereknél is, nem csak muslincáknál, akiknek nincsenek emésztőenzimjeik, amelyek a felszívódás előtt visszabontanák a pantetin egészét pantoténsavvá. A PKAN-tól szenvedő gyerekek érdekében reméljük hát, hogy ezt is meg fogják vizsgálni mihamarabb…
Bár B5-vitamin forrásként (CoA növelésre), mint láthattuk, a pantetin nem jobb, sőt picit még rosszabb is, mint pl. a Ca-pantotenát, azonban más célra nagyon is használható vegyületnek tűnik, de csakis óriási, farmakológiai/szuprafiziológiás dózisokban szedve, azaz nagyjából 1000 mg-os nagyságrendben szedve naponta. Ilyen dózisban jó hatással van a koleszterin és triglicerid szintekre, és mindenre, amire a ciszteamin nevű (természetes) gyógyszerhatóanyag is. A pantetin gyakorlatilag egy elnyújtott felszívódású ciszteaminnak tekinthető, ugyanis a belőle felszabaduló pantetein pantoténsavra és a ciszteaminra bomlik. [1,4] A B5-vitamintól eltérő hatását a ciszteaminná bomlásának köszönheti. Bár a ciszteamin hatásosabb, mint a pantetin, de sokak számára a ciszteamin nehezen tolerálható mellékhatásokat okozó gyógyszerhatóanyag, miközben a pantetin jól tolerálható bárki számára, gyakorlatilag nincs mellékhatása. [4] Ciszteamin helyettesítésére (pl. cisztin metabolizmus rendellenessége esetén) vagy a koleszterinszint szabályozására a pantetin kiváló hatóanyag. B5-vitaminnak használva azonban csak 10 x drágább, mint a sokat vizsgált Ca-pantotenát, de semmivel nem jobb.
Összefoglalva: A pantetinra nem kellene B5-vitamin forrásként tekinteni, mert bár B5-vitamin forrás, ilyen célra használni (CoA szint fokozására) hajszálnyit rosszabb, mint a jól bevált Ca-pantotenát. A ciszteamin mellékhatásoktól mentes alternatívájaként érdemes tekinteni rá. Napi 1000 mg körüli dózisban alkalmazva vérzsír/koleszterin szabályozó hatással bír egyebek mellett. Alacsonyabb dózisban alkalmazva azonban csak B5-vitamin forrásként funkcionál, de arra picit kevésbé jó, és kb. 10 x drágább (a Ca-pantotenát eleve drága, azaz ha pantetinre van cserélve egy termékben, annak fogyasztói ára feleslegesen és nagymértékben megemelkedik).
Felhasznált szakirodalom
(A bennük lévő releváns szöveget is mutatjuk, akit érdekel és nem szeretné végig bogarászni őket)
1. Czumaj A, Szrok-Jurga S, Hebanowska A, Turyn J, Swierczynski J, Sledzinski T, Stelmanska E. The Pathophysiological Role of CoA. International Journal of Molecular Sciences. 2020; 21(23):9057. https://doi.org/10.3390/ijms21239057
Abstract
The importance of coenzyme A (CoA) as a carrier of acyl residues in cell metabolism is well understood. Coenzyme A participates in more than 100 different catabolic and anabolic reactions, including those involved in the metabolism of lipids, carbohydrates, proteins, ethanol, bile acids, and xenobiotics. However, much less is known about the importance of the concentration of this cofactor in various cell compartments and the role of altered CoA concentration in various pathologies. Despite continuous research on these issues, the molecular mechanisms in the regulation of the intracellular level of CoA under pathological conditions are still not well understood. This review summarizes the current knowledge of (a) CoA subcellular concentrations; (b) the roles of CoA synthesis and degradation processes; and (c) protein modification by reversible CoA binding to proteins (CoAlation). Particular attention is paid to (a) the roles of changes in the level of CoA under pathological conditions, such as in neurodegenerative diseases, cancer, myopathies, and infectious diseases; and (b) the beneficial effect of CoA and pantethine (which like CoA is finally converted to Pan and cysteamine), used at pharmacological doses for the treatment of hyperlipidemia.
CoA Synthesis
The substrates for CoA-SH biosynthesis are Pan, cysteine and ATP. There are three main sources of blood-circulating Pan: (a) food; (b) gut microbiota; and (c) extracellular products of CoA-SH degradation of damaged cells and recycled cellular content (exocytosis) [23,24,25,26]. In the diet, CoA-SH is the main source of Pan (approx. 85% of dietary Pan is in the form of CoA-SH or phosphopantetheine), which is hydrolyzed in the intestine, and Pan, which is thus formed, is then absorbed [26,27,28]. In mammals, Pan is absorbed in the intestine and transported into cells through sodium-dependent multivitamin transporter (SMVT) [23,27]. Pan delivered into the bloodstream is transported throughout the body (to organs) by red blood cells [29].
The first step of CoA-SH biosynthesis is the phosphorylation of intercellular Pan to 4′-phosphopantothenate (PPan) by pantothenate kinase (PANK). Then PPan is condensed with cysteine by phosphopantothenoylcysteine synthetase (PPCS), followed by decarboxylation by phosphopantothenoylcysteine decarboxylase (PPCDC) to generate 4′-phosphopantetheine (PPanSH) [30]. Human PPCS utilizes ATP to activate substrate in a ligation reaction. An acyl adenylate intermediate is formed, followed by release of pyrophosphate, and binding of cysteine, and then, the final products, 4′-phosphopantothenoylcysteine and AMP, are formed [31]. In mammals, in contrast to other organisms, the last two steps in the CoA-SH biosynthetic pathway are catalysed by CoA synthase (COASY), which exhibits two enzymatic activities: 4′-phosphopantetheine adenyltransferase (PPAT) and a dephospho-CoA kinase (DPCK) [30]. PPanSH is first converted to dephospho-CoA (dPCoA) and subsequently phosphorylated to form CoA-SH. The CoA-SH synthesis pathway is presented in Figure
2. CoA Degradation
Degradation of CoA-SH may be an extracellular (intestinal or systemic) or intracellular process [23]. The extent of CoA-SH degradation depends on the supply of CoA-SH in the food and physiological state of the organism, as it determines CoA-SH usage in different metabolic processes [31].
2.2.1. Extracellular Degradation of CoA (Known as Intestinal or Systemic)
The CoA-SH degradation pathway is presented in Figure 2 and Figure 3. This pathway is catalyzed by the membrane or soluble enzymes, and not all of them are specific for CoA degradation. In systemic degradation they can hydrolyze not only the CoA-SH but also different types of acyl-CoA. However, it is not well known at which step of the acyl-CoA degradation process, the acyl group is removed [37]. The degradation of CoA-SH to pantetheine (PanSH) and finally to Pan, which is used as a substrate for intracellular CoA-SH synthesis, is a sequence of reactions including dephosphorylations and removal of nucleotide moiety (Figure 2). One of the first steps is the hydrolysis of diphosphate bond in CoA-SH, with the release of PPanSH, catalyzed by the alkaline ectonucleotide pyrophosphatase/phosphodiesterase (ENPP). Those are the promiscuous enzymes, which break down the 5′-phosphodiester bonds in nucleotides and their derivatives, may use not only the CoA-SH but also dPCoA as a substrate (dPCoA is preferred) [38,39].
In the intestinal degradation of CoA-SH the first step is most likely catalyzed by alkaline phosphatase (AP), an enzyme highly abundant in the intestinal lumen [25]. The product of this reaction, dPCoA, is later hydrolyzed by ENPP with the release of 5′-AMP and PPanSH. In systemic degradation, ENPP hydrolyzes the phosphodiester bond in CoA-SH, transforming CoA-SH into PPanSH and producing 3′,5′-ADP. Two ENPP isoforms (1 and 3) are probably involved in this process. Both are transmembrane glycoprotein homodimers, but some soluble forms have also been detected [40,41]. PPanSH released from CoA-SH or dPCoA is then dephosphorylated by AP or other unknown, phosphatases to PanSH.
The last step of the extracellular CoA-SH degradation pathway is catalyzed by a specific regulatory enzyme—pantetheinase, also known as vanin (VNN). Pantheteinase produces Pan and cysteamine from PanSH by specific cleavage of an amide bond, thus providing Pan as a precursor for intracellular CoA-SH synthesis [42]. Two isoforms (VNN1 and VNN3) are present in mice, and three isoforms are present in humans (VNN1-3) [43,44,45]. VNN1 is the most widely expressed GPI-anchored ectoenzyme. It is found in the membranes of epithelial cells, including ileal enterocytes and kidney proximal tubule epithelial cells. An additional soluble form secreted from hepatocytes has also been detected [46,47,48]. VNN3 is found in various tissues, and this isoform is not attached to membranes, and its expression is upregulated in the inflammatory state or during oxidative stress. Together VNN1 and VNN3 contribute to extracellular pantetheine degradation [44,49].
2.2.2. Intracellular Degradation of CoA
The steps of intracellular CoA-SH degradation are similar to the extracellular process. However, there are some differences, with the greatest being in the final step, when PPanSH, and eventually PanSH, is produced. Because of the lack of VNN, Pan is not produced in the intracellular process. In the cell, CoA-SH is degraded in many organelles, such as mitochondria, peroxisomes, and lysosomes. Some steps are undertaken in the cytosol.
In the mitochondrial matrix, CoA-SH and acyl-CoA are degraded by nudix (nucleoside diphosphate linked moiety X)-type motif (NUDT8) to PPanSH or acyl-4′-P-pantetheine, respectively, with the release of 3′,5′-ADP [50]. The family of NUDT hydrolases contains enzymes with a high affinity for acyl-CoA. Along with mitochondrial NUDT8, two other NUDT hydrolases (NUDT7 and NUDT19) participate in the intracellular CoA-SH degradation process. They differ not only in subcellular localization but also in a regulation pattern, size, and tissue distribution [50,51,52]. Mitochondrial NUDT8 is widely distributed among tissues. In mice, the highest amounts of NUDT8 are found in mitochondria of kidneys, heart, brown adipose tissue, liver, brain, heart, and skeletal muscles. NUDT8 hydrolyzes CoA-SH and short- and medium-chain acyl-CoA to acyl-4′-P-pantetheine [50]. The fate of the acyl-PPanSH produced in mitochondria is currently unknown. Possibly it is degraded by ACOT, but this remains speculative since there is no evidence.
Acyl-CoA degradation in peroxisomes is similar to that in the mitochondrial pathway. NUDT7 and NUDT19 are involved. NUDT7 is active against short- and medium-chain acyl-CoAs, and this activity is higher than that against CoA-SH. In mouse liver, its expression is regulated by feeding-fasting cycles, probably through PPARα action [50,51,52,53]. NUDT19 in mice is found mostly in kidneys. Its main substrate is CoA-SH, but it also shows high activity against some short- and medium-chain acyl-CoAs [52,54]. As in mitochondria, the acyl-CoAs are degraded to acyl-4′-P-pantetheine. Based on unpublished experimental results, Hunt et al. suggest that the recombinant mouse ACOT3 and ACOT8 both hydrolyze lauryl-PPanSH [55]. Antonenkov and Hiltunen suggest that the removal of fatty acids from acyl-4′-P-pantetheine could allow the PPanSH to exit peroxisomes, and enter the cytosol through a specific transporter, or freely through the membrane [56]. However, there is no clear evidence shows that PPanSH, whether produced from free CoA or acyl-CoA, reaches the cytosol [23].
Lysosomal degradation of acyl-CoA is different from peroxisome and mitochondrion degradation (Figure 3). Under acidic conditions, lysosomal acidic phosphatase 2 (LAP2) removes the phosphate group from either CoA-SH or acyl-CoA, producing dephospho-acyl-CoA [57]. In contrast to other organelles, in lysosomes, long-chain acyl-CoAs are degraded. LAP2 cooperates with palmitoyl-protein thioesterase (PPT) [58,59,60]. This cooperation leads to the final lysosomal product, which is dPCoA. There is no evidence for the existence of a lysosomal dPCoA transporter, but some putative candidate proteins have been found [61].
Some evidence suggests that a small pool of PPanSH could be further degraded to PanSH by an unknown phosphatase. The most likely candidate seems to be pantothenate kinase 4 (PANK4), a bifunctional enzyme inactive as a kinase but with weak phosphatase activity towards PPanSH [25].
Pantethine, a stable form of pantetheine (two molecules of pantetheine linked by a disulfide bridge) as a dietary supplement (used at a pharmacological dose of 600–1200 mg per day), lowers elevated levels of total cholesterol, LDL-cholesterol, triacylglycerol, and non-HDL-cholesterol concentrations [186,187,188]. The effect of orally administered pantethine also results in: (a) an increase in HDL cholesterol concentration; and (b) normalization of apolipoprotein B (apo B) and apolipoprotein A (apoA); however, the effect depends on the type of dyslipidemia [189,190,191].
Some data suggest that CoA-SH (used at 400 U per day) can improve blood triacylglycerol and lipoprotein concentrations (total cholesterol and non-HDL cholesterol were significantly reduced, and HDL cholesterol was increased) to a greater extent than pantethine (used at 600 U per day) [192].
Taken together, the results published thus far indicate that CoA-SH and pantethine can be useful in lowering elevated levels of circulating lipids in some diseases. This effect is most impressive when pantethine side effect and toxicity (practically none, when used at concentrations effectively lowering blood lipid concentration) are compared with commonly used drugs that lower circulating lipids (for instance, statins). However, the effect of CoA-SH or pantethine on circulating lipids is relatively slow. Usually, a maximal effect is observed at 4 months but may take up as long as 6–9 months.
The exact molecular mechanism of action of CoA-SH and pantethine on blood lipid concentration is unknown. Although pantethine is a precursor for vitamin B5 synthesis and intake of the pharmacological dose of pantethine results in higher circulating vitamin B5 concentration, the production of vitamin B5 is not the mechanism of action because intake of panthotenic acid does not have the same effect on serum lipid concentration [2]. Pantethine-induced inhibition of acetyl-CoA carboxylase by cysteamine, the product of pantethine and CoA degradation, and inhibition of HMG-CoA reductase and cholesterol synthesis in isolated hepatocytes by pantethine may explain, at least in part, the fact that pantethine (and possibly CoA-SH) administration in pharmacological doses is effective in reducing plasma triacylglycerol and cholesterol (total cholesterol, LDL-cholesterol, and non-HDL-cholesterol) concentrations [193,194,195].
Pantethine (specifically, the cysteamine formed from pantethine) inhibition of acetyl-CoA carboxylase decreases the level of malonyl-CoA, which is (a) a substrate for fatty acid synthesis; and (b) an allosteric inhibitor of CPT1, a key regulator of fatty acid oxidation. Consequently, this reduction of malonyl-CoA leads to (a) a decrease in fatty acid synthesis; and (b) an increase in fatty acid oxidation in mitochondria. In turn, plasma lipids are affected, especially by triacylglycerol-lowering effects.
Although several clinical trials have shown that CoA-SH and especially pantethine used at pharmacological doses reduce circulating lipid levels in patients with dyslipidemia associated with different pathologies, it seems that additional studies are necessary to determine whether CoA-SH or pantethine supplementation has a beneficial effect on cardiovascular risk markers independently of or in combination with a healthy diet. Moreover, further research is also needed to explain the exact molecular mechanism of CoA-SH and pantethine action on circulating lipid concentration. At present, it is safe to say that administration of CoA-SH is not entirely different from the administration of pantethine because in the end both compounds are converted to Pan and cysteamine.
2. Chang X, Zhang J, Jiang Y, Yao B, Wang J, Wu Y. Pilot trial on the efficacy and safety of pantethine in children with pantothenate kinase-associated neurodegeneration: a single-arm, open-label study. Orphanet J Rare Dis. 2020;15(1):248. Published 2020 Sep 14. doi:10.1186/s13023-020-01530-5
Pantethine is a marketed drug used to treat hyperlipidemia. Studies on a PANK2 knockout Drosophila model [6] and a PANK2 knockout mouse model [7] showed that pantethine could aid mitochondrial dysfunction, increase CoA levels in tissues, and rescue the neuromuscular phenotypes. In this study, we aimed to explore the efficacy and safety of the re-purposed drug treatment of pantethine in children with PKAN.
The study lasted for 24 weeks. Patients received pantethine through oral administration (capsules, 450 mg per serving, Jarrow Formulas, USA). The dose started at 20 mg/kg per day, gradually increased to 60 mg/kg per day within 4 weeks and maintained at 60 mg/kg per day (divided into two dosage) until the end of the study.
The serum level of CoA at the baseline and W24 were 26.1 (9.3–122.4) nmol/mL and 14.3(16.5–162.0) nmol/mL, respectively. No significant difference was found before and after 24 weeks of treatment (Z = 1.014, P = 0.31).
We measured the concentration of pantethine and sodium pantothenate in the plasma. We could only detect sodium pantothenate but not pantethine, which is because that pantethine was rapidly hydrolyzed to pantothenic acid and cysteamine [8].
3. Yoshii K, Hosomi K, Sawane K, Kunisawa J. Metabolism of Dietary and Microbial Vitamin B Family in the Regulation of Host Immunity. Front Nutr. 2019;6:48. Published 2019 Apr 17. doi:10.3389/fnut.2019.00048
Vitamin B5 is found in high concentrations as CoA or phosphopantetheine in liver, eggs, chicken, and fermented soybeans. CoA and phosphopantetheine are converted to free pantothenic acid by endogenous enzymes such as phosphatase and pantetheinase in the small intestine. Free pantothenic acid is absorbed via sodium-dependent multivitamin transporter (SMVT) expressed on the epithelium of the small intestine and is then released into the blood (80). Finally, free pantothenic acid is converted back to CoA and distributed throughout the body, particularly to the liver and kidney.
4. Wittwer CT, Gahl WA, Butler JD, Zatz M, Thoene JG. Metabolism of pantethine in cystinosis. J Clin Invest. 1985;76(4):1665-1672. doi:10.1172/JCI112152
Abstract: D-Pantethine is a conjugate of the vitamin pantothenic acid and the low-molecular-weight aminothiol cysteamine. Pantethine is an experimental hypolipemic agent and has been suggested as a source of cysteamine in the treatment of nephropathic cystinosis. We treated four cystinotic children with 70-1,000 mg/kg per d oral D-pantethine and studied its metabolism. Pantethine was rapidly hydrolyzed to pantothenic acid and cysteamine; we could not detect pantethine in plasma after oral administration. The responsible enzyme, “pantetheinase,” was highly active in homogenates of small intestinal mucosa and plasma. The Michaelis constant of the rat intestinal enzyme was 4.6 microM and its pH profile showed a broad plateau between 4 and 9. Pantothenate pharmacokinetics after orally administered pantethine followed an open two-compartment model with slow vitamin elimination (t1/2 = 28 h). Peak plasma pantothenate occurred at 2.5 h and levels over 250 microM were seen at 300 times normal. Apparent total body storage of pantothenate was significant (25 mg/kg), and plasma levels were elevated threefold for months after pantethine therapy. Plasma cysteamine concentrations after pantethine were similar to those reported after equivalent doses of cysteamine. However, at best only 80% white blood cell cystine depletion occurred. We conclude that pantethine is probably less effective than cysteamine in the treatment of nephropathic cystinosis and should only be considered in cases of cysteamine intolerance. Serum cholesterol was decreased an average of 14%, which supports the potential clinical significance of pantethine as a hypolipemic agent. Rapid in vivo hydrolysis of pantethine suggests that pantothenate or cysteamine may be the effectors of its hypolipemic action.
5. National Institute of Health, Pantothenic acid Fact Sheet for Health Professionals
Link: https://ods.od.nih.gov/factsheets/PantothenicAcid-HealthProfessional/
About 85% of dietary pantothenic acid is in the form of CoA or phosphopantetheine [2,4]. These forms are converted to pantothenic acid by digestive enzymes (nucleosidases, peptidases, and phosphorylases) in the intestinal lumen and intestinal cells. Pantothenic acid is absorbed in the intestine and delivered directly into the bloodstream by active transport (and possibly simple diffusion at higher doses) [1,2,4]. Pantetheine, the dephosphorylated form of phosphopantetheine, however, is first taken up by intestinal cells and converted to pantothenic acid before being delivered into the bloodstream [2]. The intestinal flora also produces pantothenic acid, but its contribution to the total amount of pantothenic acid that the body absorbs is not known [4]. Red blood cells carry pantothenic acid throughout the body [4]. Most pantothenic acid in tissues is in the form of CoA, but smaller amounts are present as acyl carrier protein or free pantothenic acid [1,4].
6. Scientific Opinion of the Panel on Food Additives and Nutrient Sources added to Food (ANS) on a request from the Commission on pantethine as a source for pantothenic acid added as a nutritional substance in food supplements. The EFSA Journal (2008) 865, 1-20.
Link: https://efsa.onlinelibrary.wiley.com/doi/pdf/10.2903/j.efsa.2008.865
Ingested pantethine is reduced to pantetheine with reduced glutathione (GSH) as cofactor (Durr and Cortas, 1964). One equivalent of pantethine results in two equivalents pantetheine in the intestinal lumen. Pantetheine is in turn absorbed from the intestinal lumen by mucosa cells (van den Berg, 1997; Bender, 2003). Although in earlier studies simple diffusion was reported to be the main transport system of pantetheine, there is now ample evidence that transport is affected in mammals through a saturable, sodium dependent transport system in the jejunum (SCF, 2002; Bender, 2003). In the intestinal mucosa cells pantetheine is hydrolysed rapidly to pantothenic acid by pantotheinase (Bender, 2003). The absorption and distribution of pantethine was studied by Tachizawa et al. (1979) using jejunum and ileum rat intestinal loops. The results indicated that pantethine is absorbed from the intestine in its unmodified form and as pantothenic acid. 14C-labelled pantethine was given at a dose of 200 mg/kg bw orally to rats, and two hours after the administration, radioactivity was detected in whole blood, aorta, kidney and liver. In another study the changes in blood concentration and the urinary excretion of free and bound pantothenic acid were studied after oral and intravenous administration of pantethine (21.6 µmoles/kg equivalent to 43.2 µmoles pantothenic acid equivalents/kg) to rats (Ono et al., 1974). The results were compared with those obtained after administration of calciumpantothenate (Ca-pantothenate, 21.6 µmoles/kg). The concentration of total pantothenic acid in blood expressed in nmoles pantothenic acid equivalents/ml reached its maximum levels 2-4 hours after administration and amounted at 2 hours after administration to 2.52 + 0.03 nmoles pantothenic acid equivalents/ml for the control, 4.06 + 0.16 nmoles pantothenic acid equivalents/ml for the pantethine group and 3.43 + 0.07 nmoles pantothenic acid equivalents/ml for the Ca-pantothenate group. Thus, the concentration of total pantothenic acid in blood was statistically significantly but only 18% higher in the pantethine group than in the Ca-pantothenate group after oral administration of vitamins, whereas on the basis of pantothenic acid equivalents the dose of pantethine was 2- fold higher than that of pantothenic acid. The excretion rates of total pantothenic acid in 24- hours urines were 29±3% and 18±2% of the dose rates of the pantethine and Ca-pantothenate groups, respectively. The authors conclude that these findings indicate that pantethine is more absorbable through the gastrointestinal wall of the rat than Ca-pantothenate. No significant difference was, however, found in the amounts of bound pantothenic acid in blood between these two groups. Pantethine was found to be converted to some extent to pantothenic acid by an enzyme during passing the intestinal mucosa. After an intravenous injection of pantethine, about 50% of total pantothenic acid in blood was pantethine and the rest was free pantothenic acid, while little bound pantothenic acid was detected after injection of Ca-pantothenate. The decrease in total pantothenic acid in blood was significantly delayed in the early period after injection in the pantethine group when compared with that of Ca-pantothenate group, although both compounds were almost completely eliminated in 24 hour urines after injection (Ono et al., 1974).
Data in the literature indicate that pantethine is about equally rapidly absorbed as calcium pantothenate when given orally to rats (Ono et al., 1974) with plasma levels being at their maximum values already upon 2 hours post dosing and only slightly (18%) higher in animals dosed with pantethine at a dose that was twice as high as that of calcium pantothenate based on pantothenic acid equivalents. Based on this information the Panel concludes that the bioavailability of pantothenic acid from pantethine upon oral intake might be comparable to or lower than that of pantothenic acid.